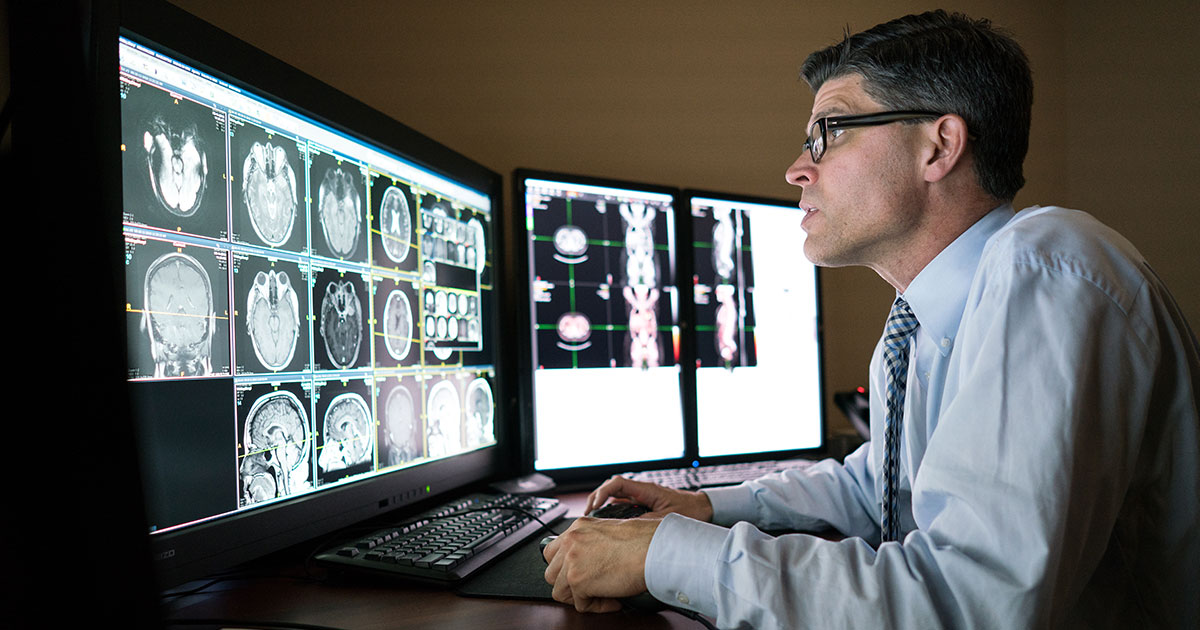
Radiation therapy is a common component of cancer care. The National Cancer Institute estimates that more than half of all cancer patients are prescribed radiation at some point during their treatments. Most of the time, the therapy involves directing a radiation beam at a tumor to try to kill the cancerous growth. More recently, oncologists have shown increasing interest in radiopharmaceuticals—radioactive particles attached to drugs that precisely target and kill cancer cells.
“Some radiopharmaceuticals are chemically targeted so they are attracted to something like damaged bone, or a certain target chemical, or they are an isotope only certain cells would want to take in,” says Sean Cavanaugh, MD, National Director of Radiation Oncology for Cancer Treatment Centers of America® (CTCA). “Because of this, they are very useful for disease that is widespread or disease that we cannot completely image.”
The potential of such treatment has been known for decades, ever since the use of iodine in the 1940s to treat thyroid cancer. But it has taken time for radiopharmaceuticals to emerge as real-world cancer treatments.
“We went from one to two in 70 years, and then from two to three in last three years. We are going to see many more over the next five to ten years,” says Jason Beland, MD, National Director of Radiology for CTCA©.
The global market for radiopharmaceuticals, which reached $6.1 billion in 2019, is expected to double to $12.6 billion by 2027, according to a December article in the American Journal of Managed Care®. Radiopharmaceuticals appear to have fewer side effects than traditional radiation therapy and are better equipped to target cancer cells. Targeted radiopharmaceutical therapy, also called molecular radiation therapy, may be effective on both isolated tumors and on metastatic cancer that has spread throughout the body.
How radiopharmaceuticals work
Radiopharmaceutical therapy builds on the progress made in the precision medicine fields of targeted therapy and immunotherapy. Targeted therapy and immunotherapy are designed to attach to specific receptors on cancer cells or nonmalignant cells near a cancerous tumor. In immunotherapy, the connections induce the body’s own immune system to fight the cancer. In targeted therapy, monoclonal antibodies work to kill cancer cells and stop a tumor from growing or spreading.
In targeted radiopharmaceutical therapy, a monoclonal antibody or other drug carries a radioactive isotope that will kill off cells—both cancer and healthy cells—in its immediate vicinity. This targeted approach differs from a more traditional external beam radiation therapy (EBRT), where the radiation is coming from outside the body.
“Think about everything on the way in and the way out,” when thinking about traditional radiation treatment, Dr. Cavanaugh says. “If we are targeting something in the middle of the body, the pancreas let’s say, we have to go through quite a bit of tissue to get there and then there’s quite a bit on the path on the way out.”
While the amount of damage to healthy cells may be reduced with radiopharmaceuticals, there is still some risk to other parts of the body as the radioactive drug flows through the bloodstream before being expelled in the urine, Dr. Beland says. “We are injecting it into a vein, it travels around the body, about 20 percent of your blood volume goes through your kidneys every minute, things that are not needed spill out into the urine and bladder and is subsequently urinated out,” Dr. Beland says.
Targeted radiopharmaceutical therapy is similar to another form of targeted therapy where monoclonal antibodies carry a chemotherapeutic drug to kill cancer cells. Some researchers say treatments using radiopharmaceuticals may be most beneficial in conjunction with more traditional radiation therapy. External radiation may be best for killing off a concentrated tumor, while radiopharmaceuticals may better address and catch metastases elsewhere in the body.
From iodine to monoclonal antibodies
Radiopharmaceuticals are drugs that contain radioactive materials called radioisotopes, according to the American Cancer Society. They may be injected into a vein or artery, taken orally in liquid form, or placed into a body cavity.
The earliest example of a radiopharmaceutical is the use of iodine for both imaging and treatment of thyroid cancer. The iodine primarily accumulates in the thyroid, with most of the rest passing out of the body in the urine. Radioactive iodine is often given after thyroid surgery to destroy remaining cancerous tissues.
“That one was all worked out for us. That’s why we discovered it so early. Since then, it’s gotten a lot more sophisticated,” Dr. Cavanaugh says of the development of drugs that are able to provide pathways for the radionuclide to cancerous cells.
Unlike other forms of targeted therapy, where the goal is to get antibodies to the tumor site and do their job, the radioactive isotopes have only a limited amount of time to do their damage before the cancer cells have a chance to flush them out, says Charles Kunos, MD, PhD, of the National Cancer Institute’s Cancer Therapy Evaluation Program (CTEP). As the radioactive compound decays, it releases energy that also damages the DNA of nearby cells, which may lead to their death. Cancer cells are very susceptible to radiation-induced DNA damage.
Radiopharmaceuticals and cancer
Among the radiopharmaceuticals approved by the U.S. Food and Drug Administration for treating cancers are:
- Radium-223 dichloride (Xofigo®) for metastatic prostate cancer in the bones
- Sodium iodide I-131 (Hicon®) for thyroid cancer
- Lobenguane iodine-131 (Azedra®) for adrenal gland tumors
- Lutetium-177 (Lutathera®) for neuroendocrine tumors of the digestive tract
- Yttrium-90 (Zevalin®) for non-Hodgkin lymphoma
In addition, samarium-153 lexidronam (Quadramet®) has been approved for its pain-killing properties for metastatic bone lesions in breast cancer, prostate cancer and other cancers.
Radiopharmaceutical therapy is usually delivered by radiation oncologists, nuclear medicine physicians, radiologists and some endocrinologists. Some medical experts think medical schools may need to develop a whole new field of study that combines nuclear medicine, radiation oncology and general oncology to take full advantage of the potential of radiopharmaceuticals. Medical physicists are also required to deal with the radionuclide dosimetry for proper delivery of the medications and other radiation safety issues. Multidisciplinary training is required to administer and manage the radiopharmaceuticals, according to a July 2020 article in the journal Nature Reviews Drug Discovery.
Already, medical professionals handling radiopharmaceutical therapy require certification as authorized users from the American Board of Radiology, including 700 hours of training and supervised practice using radiopharmaceuticals, Dr. Cavanaugh says. “Training must include radiobiology, handling and safety, cancer biology and medical physics,” he says.
Therapy and imaging
Radiopharmaceuticals are not used only as therapy. Using a different radionuclide that is more suitable for imaging, they can be utilized to highlight if and where certain types of cancer exist in the body. Such drugs are used when performing positron emission tomography (PET) scans. There are more than 10 radiopharmaceuticals approved for imaging.
One of the latest, a prostate-specific membrane antigen (PSMA) called Gallium 68 PSMA-11, was approved for use on Dec. 1 by the U.S. Food and Drug Administration. It is the first PET drug for imaging PSMA positive lesions in men with prostate cancer.
“I think that is a big one, because that is the most common cancer in men. There are breast agents in development. A number of other agents are in phase 2 and phase 3 clinical trials,” Dr. Cavanaugh says.
Radiopharmaceuticals are part of an approach called theranostics, which relies on a lab-produced drug to carry radioactive isotopes to specific cancer cells, sometimes attached to low-dose radiation to map a cancer and sometimes to high-dose radiation as therapy. Theranostics gives real-time information to oncologists so that treatment may be altered based on an individual patient’s cancer.
“We’re marrying those three things. The diagnostic piece, the imaging has always told us is where is the disease; the biodistribution of whatever drug we are administering; and the subsequent treatment with the ability to deliver radiation,” Dr. Beland says. “We are getting the biodistribution of drug, the dose of radiation and the diagnostic all together here.”